Alright, fine! Sometimes adding “quantum” to something does make it better— that is the case of quantum sensors. Their precision is often far beyond that of their classical counterparts because they are based on quantum mechanics instead of Newton’s laws.
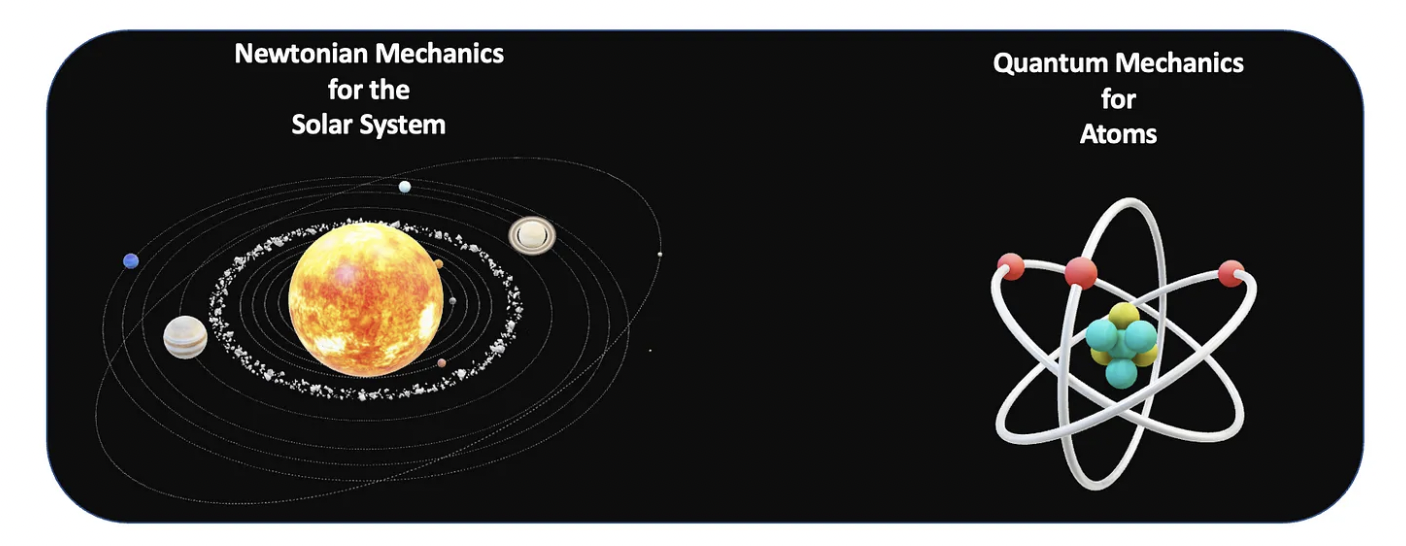
Quantum mechanics, over a century ago, had no immediate use other than explaining a few phenomena, such as the black-body radiation, the photoelectric effect and the discrete atomic spectrum. In fact, some people even thought there was nothing much left to discover in Physics anymore — I am talking about Max Planck’s professor. Since then, quantum mechanics became the most successful theory ever built by humankind, with countless practical applications, from lasers to PET scans.
However, progress was somewhat hindered by the fragility of quantum properties (e.g., superposition and entanglement), which ironically turned out to be quantum sensors’ strength. Such devices harness those fragilities to measure minute effects that are well beyond classical sensors’ sensitivities — talk about a plot twist.
In the previous articles, I focused on technical aspects of quantum computing, but here we will go over this other side of quantum technologies and why use them in the first place. I remark quantum computing itself benefits from better control of individual quantum systems, which is fundamental in quantum sensor design.
Quantum Sensors Success Stories
If you are a fan of hit backstabbing-filled TV Shows (e. g., Game of Thrones, Succession, etc.), you know information is power. But the means to obtain it matter just as much. A fundamental method of getting information is via measurement of a quantity. Most of the measurements today are made using ‘classical methods’. For example, to measure distances, one can use a laser and a precise clock. Unless we are talking about atomic clocks, this is an example of a classical measurement — This is how we measure the distance to the moon, for example.
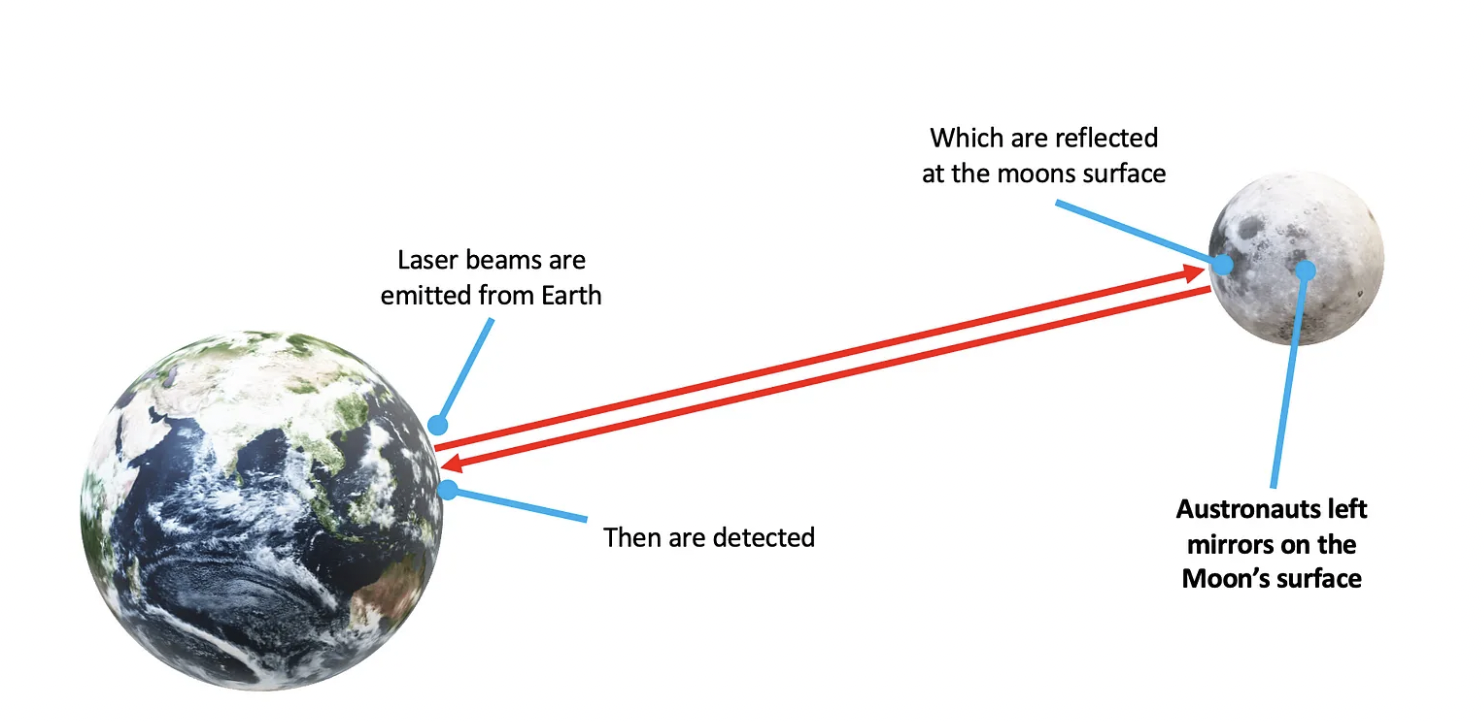
If you need increased precision, you might ask for a device with fundamentally different principles. For example, a sophisticated (and very expensive) clock may miss 6 seconds a day [1], while an atomic clock would miss 1 second in around 30 million years [2] — Not enough precision for you? Further improvements are on the way [3].
Like an atomic clock, devices that use quantum mechanics for measurements and/or probing are called quantum sensors [1]. The theoretical foundations of these sensors are an entire field of study called quantum metrology. The University of Vienna has many prominent researchers in this field. I recommend a visit to their webpage [4].
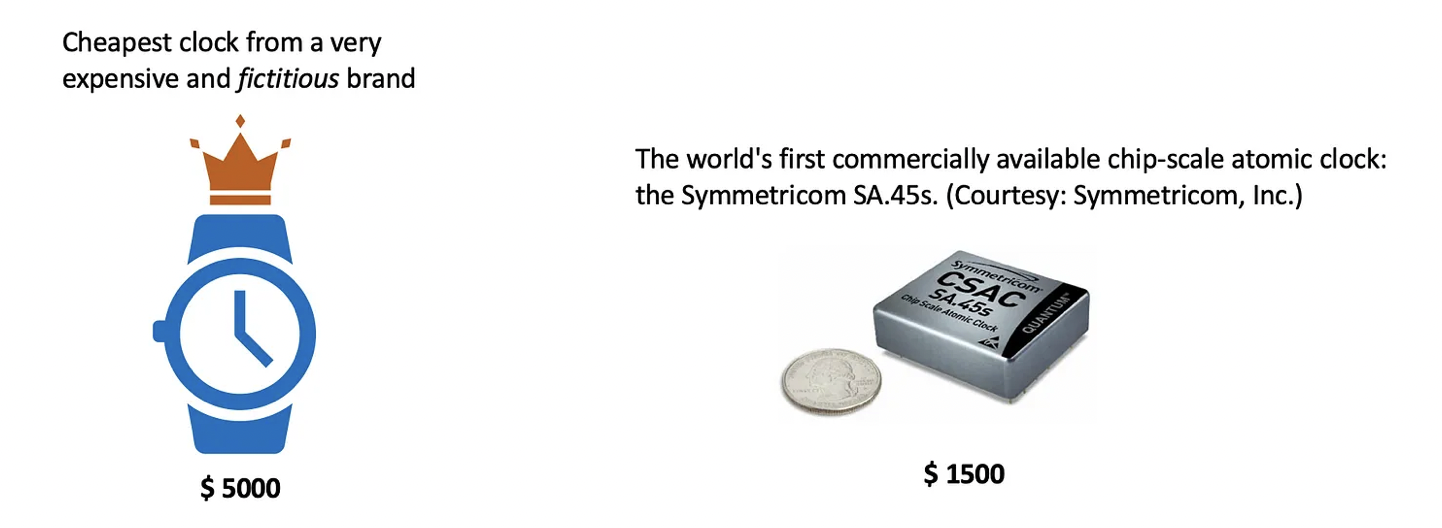
The potential of quantum sensors is difficult to overestimate. They can be used in non-invasive clinical procedures, such as scanning brain activity in detail [5, 6]. For instance, researchers used a quantum system to detect single-neuron activity in live organisms. In another remarkable feat, a 1-qubit sensor was used in nuclear magnetic resonance of single protein molecules [7, 8]. Also, we must not forget that quantum effects helped detecting gravitational waves at the Laser Interferometer Gravitational-Wave Observatory (LIGO), in arguably the most precise experiment in human history [9].
Speaking of gravity, quantum sensors can detect ore and water underground deposits, predict seismic activity and volcanic eruptions, and survey soil properties for civil engineering purposes [10] (no digging required!). In fact, a recent breakthrough illustrates this technology is very close to industrial applications.
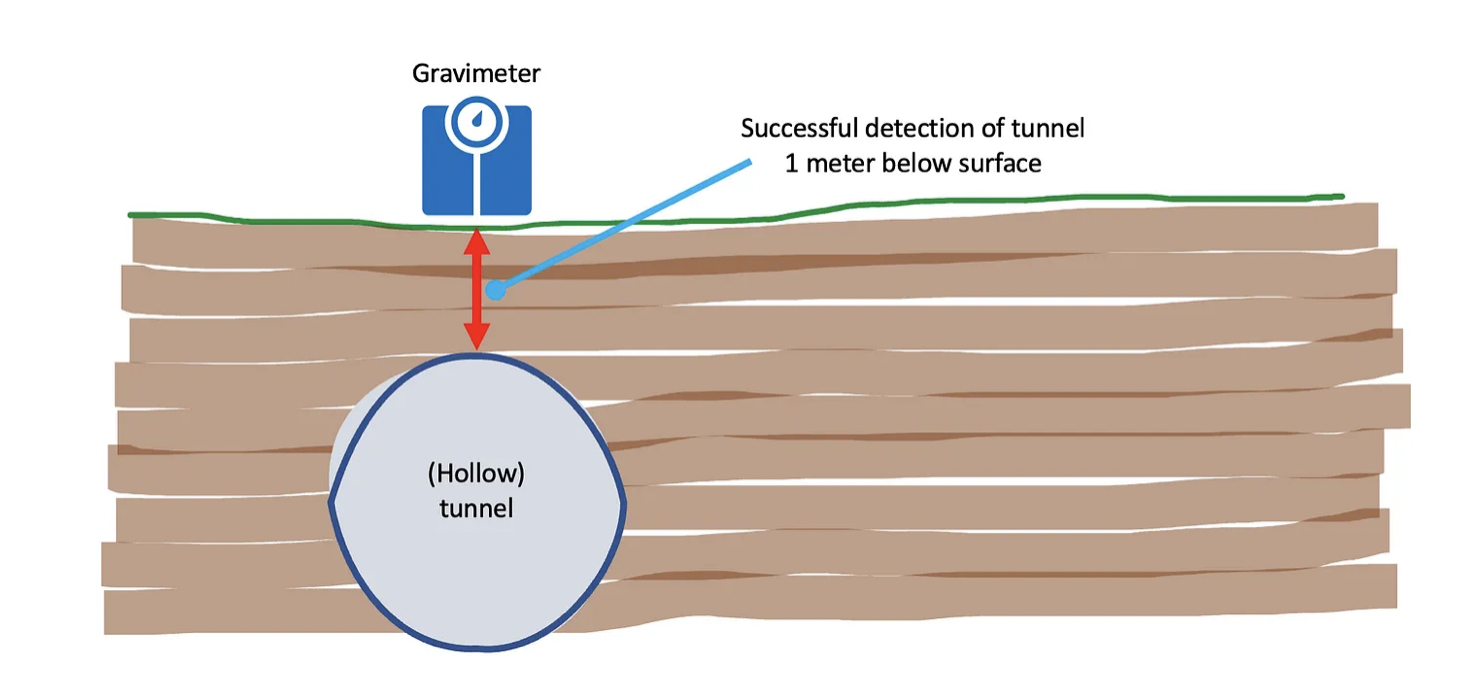
Via the same principles as gravimeters, quantum accelerometers can be used in inertial navigation technologies. In other words, by precisely measuring acceleration, one can learn about the total displacement: this essentially means a satellite-free GPS system. This type of navigation is also possible with precise knowledge and detection of the Earth’s magnetic field — a device called quantum magnetometer does just that [11]. In fact, they can also be used in closely studying the brain and soft tissues, as mentioned above. Other enabling capabilities are quantum radars and enhanced resolution [12] — in which the military has much interest, as they have the potential to detect stealth technology.
Several of the applications mentioned here are based on nitrogen-vacancy systems (just like the one in the figure below). They consist of defects in a diamond lattice that behave like a qubit, emitting and absorbing light in specific frequencies. This system is used in applications such as innovative imaging processes of 2D materials [14]. Current equipment is quite bulky, but it could be more compact, as done by the MIT [15]. On the bright side, they function at room temperature and are extremely sensitive to electric and magnetic fields. This makes such systems very versatile since virtually all signals can be translated into electric/magnetic inputs.
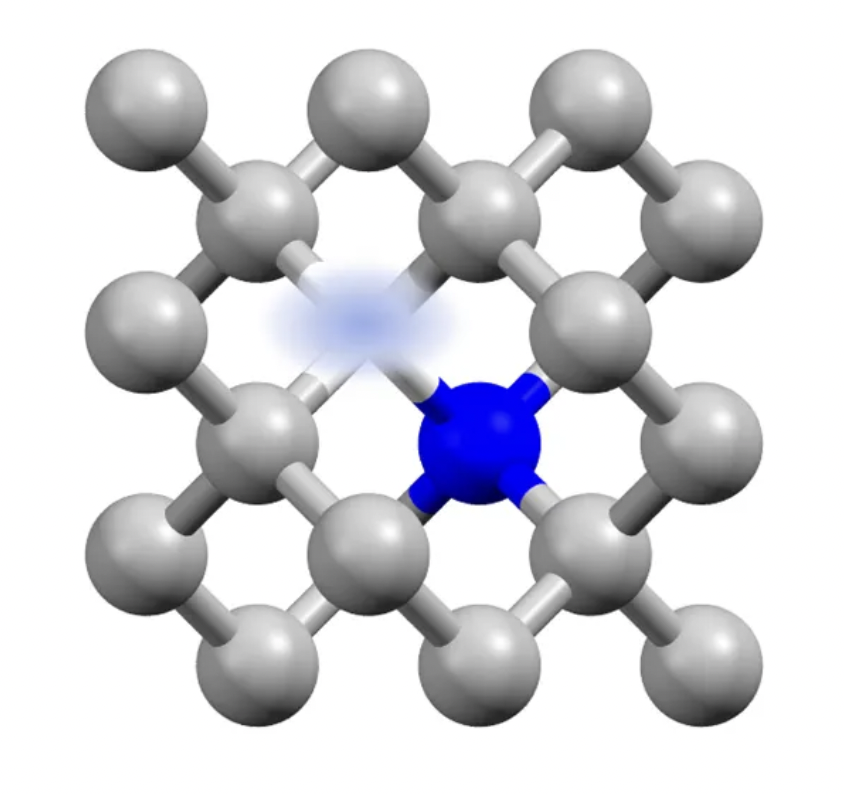
Although quantum sensors are not designed to perform complex computational tasks, the techniques developed to harness their properties have a real impact in the building of quantum computers. These techniques can be employed to isolate quantum systems to increase their coherence time, that is, the time they retains their quantum behavior. Other techniques enable manipulation of single-atom systems, such as ions or cold atoms in lattices, which is the main product offered by some quantum computer manufacturers.
Some notes for the most curious
In this section I mention a few theoretical tools used in describing quantum systems and in estimating their sensitivity. You can in principle skip it without damaging the overall flow of this article. But I recommend you try to at least skim through it. Buckle up, here we go!
Quantum sensors may rely on photons [16] or qubits of solid-state systems [17]: each is a whole research field on its own, but both rely on entanglement, interference, and squeezed coherent states to improve the precision of measurements [18].
For photon-based sensors, one needs an in-depth knowledge of continuous variable systems and their evolution, both isolated (i.e., cut off from the environment) and open (i.e., in contact with an external environment). In photonic systems, the noise can be dramatically reduced using squeezed modes, so one needs to learn multi-mode squeezing.
Among the most important applications are optomechanical systems (quantum systems, in the form of a mechanical oscillator in an optical cavity), such as the one used in [12]. Such systems are sensitive to minute variations in gravity. They could revolutionize earth sciences, engineering and ground-recognition for the military. However, there is much room for improvement regarding their readout, fidelity and other quality factors [19].
As benchmarks, it is useful to evaluate the Classical and Quantum Fisher Information (CFI, QFI), and the Cramer-Rao inequality (CRI) [20]; they provide limits on the sensibility and precision of the sensors based on these quantum systems.
Concerning the solid state-based quantum sensors, the tools are somewhat easier to learn, especially because the systems have finite dimension. However, this does not imply these sensors are less capable. Accordingly, one must learn how to evolve systems with multiple qubits and how to model their interactions. In principle, linear algebra, statistical analysis, and knowledge on open quantum systems would provide the basics.
Now let us go beyond the underlying theory and wonder a bit about other possible applications of quantum sensors.
Speculating applications for quantum sensors
Here are some speculations regarding near-term and future applications of quantum sensors:
- Use gravimeters to understand city soil structure. That may help reduce floods and allow smarter design of roads and urban buildings. It may also enable detection of water deposits for their preservation and conscious use.
- Find underground structures to retrieve historically relevant structures/artefacts — too farfetched?
- Quantum sensors for quality control. Could quantum sensing techniques improve current methods, such as electron microscopy?
- Some systems are extremely sensitive to electric/magnetic fields, temperature, strain etc. Can we come up with other uses by finding interesting effects that may disturb quantum systems? That way, more signal types can be detected.
- The current electron microscopy methods may not be employed in sensitive (e.g., biological) molecules. Maybe other quantum sensors can be used with no damage to such fragile molecules.
- In [22], researchers write that seeing inside a battery coin cell is not possible with electron spectroscopy. Understanding the internal degradation of batteries will likely have a positive impact in their design. Maybe quantum sensors can give us a better idea of what is going on, and eventually help building better batteries.
PS: if any of the readers founds a successful company with any of these ideas, I want 10% of the company shares, thank you.
Some leading companies in quantum sensing tech
This subject already finds numerous applications in industries, and a few companies already commercialize products. Among them are:
• μQuans (France) specializes in gravity measurements: www.muquans.com
• Qnami (Switzerland) builds nitrogen- vacancy-based quantum sensors for magnetic fields, with many imaging applications: www.qnami.ch
• Thales (France) is also in the game, but their website highlights other non-quantum applications: www.thalesgroup.com
• AOsense (USA), focuses on quantum gyroscopes for navigation purposes: www.aosense.com
At ColibrITD our goal is bringing Quantum Computing for All. We use the available universal quantum computers to obtain the most quantum advantage for our customers’ use cases. In that quest, we study the building blocks of quantum computers and learn of their other potential uses.
References
[1] www.swisswatchexpo.com/TheWatchClub/2019/05/31/tips-for-improving-rolex-accuracy/
[2] https://en.wikipedia.org/wiki/Atomic_clock
[3] Entanglement on an optical atomic-clock transition. E. Pedrozo-Peñafiel et al. Nature 588 (2020) 414. https://www.nature.com/articles/s41586-020-3006-1
[4] https://www.iqoqi-vienna.at/research/huber-group/quantum-metrology
[5] https://lejournal.cnrs.fr/articles/les-capteurs-lautre-revolution-quantique
[6] Non-invasive detection of animal nerve impulses with an atomic magnetometer operating near quantum limited sensitivity. K. Jensen et al. Scientific Reports 6(2016) 29638. https://www.nature.com/articles/srep29638
[7] Nuclear magnetic resonance detection and spectroscopy of single proteins using quantum logic. I. Lovchinsky et al. Science 351 (2016) 836. https://science.sciencemag.org/content/351/6275/836
[8] Quantum sensing with arbitrary frequency resolution. J. M. Boss, K. S. Cujia, J. Zopes, C. L. Degen. Science 356 (2017) 837. https://science.sciencemag.org/content/356/6340/837
[9] Observation of Gravitational Waves from a Binary Black Hole Merger. B. P. Abbot et al. (LIGO and VIRGO Collaborations). Physical Review Letters 116(2016) 061102. https://journals.aps.org/prl/abstract/10.1103/PhysRevLett.116.061102
[10] A French company, Muquans, is specialized in quantum gravimeters. Find more at: www.muquans.com
[11] Can a Quantum Nondemolition Measurement Improve the Sensitivity of an Atomic Magnetometer?. M. Auzinsh et al. Physical Review Letters 93 (2004) 173002. https://journals.aps.org/prl/abstract/10.1103/PhysRevLett.93.173002
[12] Quantum Radar. L. Maccone, C. Ren. Physical Review Letters 124 (2020) 200503. https://journals.aps.org/prl/abstract/10.1103/PhysRevLett.124.200503
[13] https://register.epo.org/application?number=EP06254079
[14] https://science.sciencemag.org/content/364/6444/935
[15] https://news.mit.edu/2019/quantum-sensing-chip-0925
https://news.mit.edu/2019/quantum-sensing-measures-magnetic-fields-0315
[16] Advances in photonic quantum sensing. S. Pirandola, B. R. Bardhan, T. Gehring, C. Weedbrook, S. Lloyd. Nature Photonics 12 (2018) 724. www.nature.com/articles/s41566-018-0301-6
[17] Quantum Sensing. C. L. Degen, F. Reinhard, P. Cappellaro. Reviews of Modern Physics 89 (2017) 035002. https://journals.aps.org/rmp/abstract/10.1103/RevModPhys.89.035002
[18] Phase sensitivity at the Heisenberg limit in an SU(1,1) interferometer via parity detection. D. Li et al. Physical Review A 94 (2016) 063840. https://journals.aps.org/pra/abstract/10.1103/PhysRevA.94.063840
[19] Cavity-enhanced microwave readout of a solid-state spin sensor. E. R. Eisenach et al. Nature Communications 12 (2021) 1357. https://www.nature.com/articles/s41467-021-21256-7
[20] Probabilistic and statistical aspects of quantum theory. A. S. Holevo. Vol. 1. Springer Science & Business Media. Berlin, 2011.
[21] Electron ptychography of 2D materials to deep sub-ångström resolution. Yi Jiang et al. Nature 559 (2018) 343. https://www.nature.com/articles/s41586-018-0298-5
[22] News feature at Nature’s website: https://www.nature.com/articles/d41586-018-07448-0
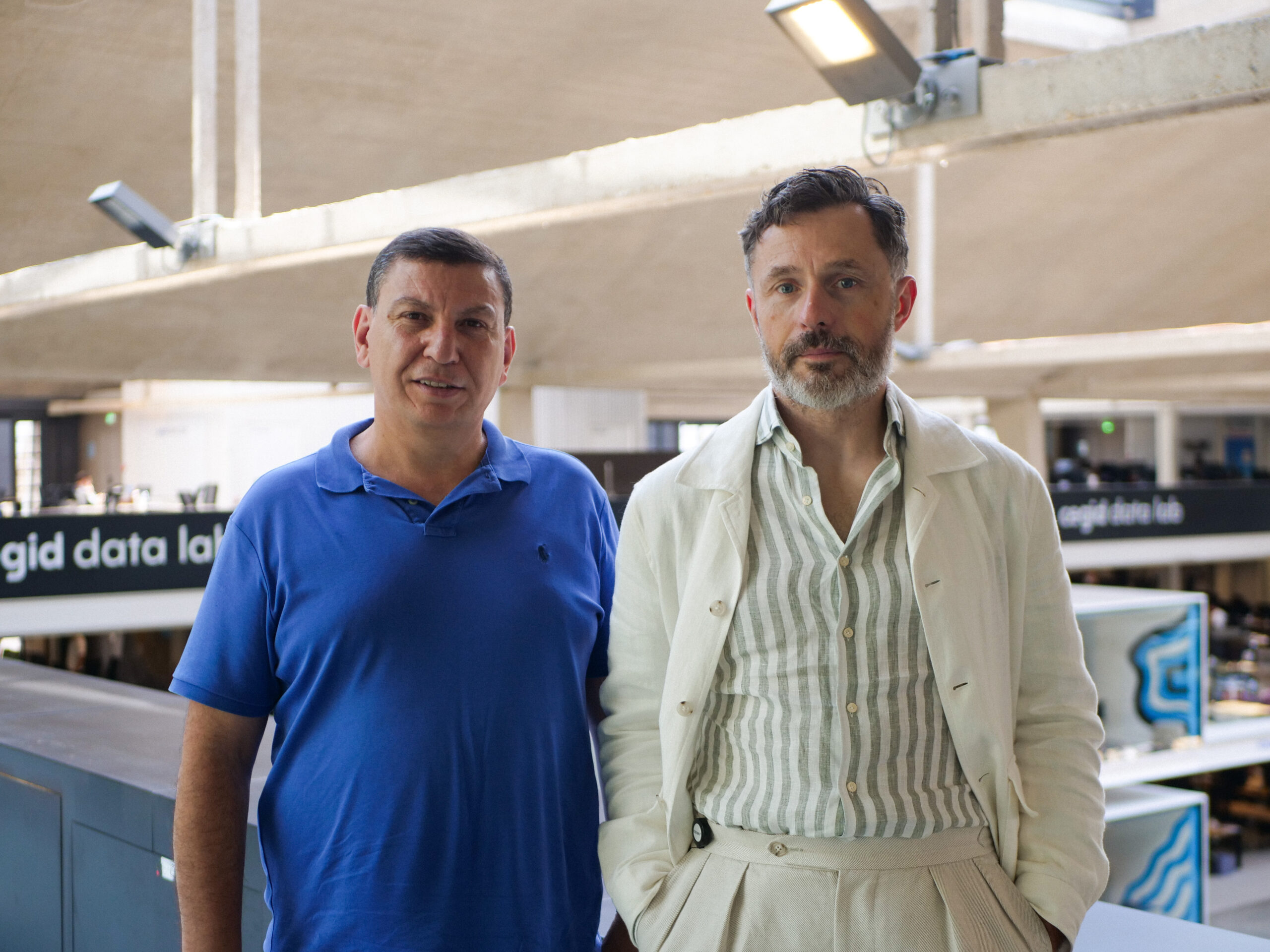